Glasses and Glass–Ceramics for Biomedical Applications
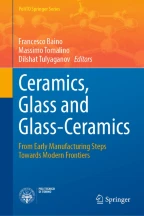
The invention of bioactive glasses has undoubtedly represented an important watershed in the history of biomedicine, innovatively revolutionizing the key concept of biomaterials. Although 50 years have passed since the first bioactive glass (45S5 Bioglass ® ), these materials still continue to inspire numerous generations of researchers all over the world, attracted by the promise of numerous possible fields of investigations given by the versatility of glass manufacturing and processing strategies. This allows obtaining final clinical products that are incredibly diverse in terms of chemical characteristics, shape and texture and, therefore, adaptable to different therapeutic needs. The possibility to tune textural properties and degradation rates, perform high-temperature sintering processes without or minimally altering the original properties of the glass, as well as the facile introduction of therapeutically active ions within the composition and the easy surface functionalization led, over year, to the development of multiple pruducts to be used in various clinical fields, including the regeneration of both hard and soft tissues, bacterial/viral infection treatments and development of antitumoral strategies. This chapter opens a wide window on the world of bioactive glasses, starting with the description of their peculiar chemical properties, discussed in relation to the most commonly used manufacturing processes to obtain glass monoliths or particles. Then, an overview on the most common applications of BG-based products will be provided, paying particular attention to porous scaffolds for bone tissue engineering, bioactive coatings, antibacterial glasses and surface functionalization. In conclusion, a comprehensive overview on clinical applications updated to the state of the art will be provided.
This is a preview of subscription content, log in via an institution to check access.
Access this chapter
Subscribe and save
Springer+ Basic
€32.70 /Month
- Get 10 units per month
- Download Article/Chapter or eBook
- 1 Unit = 1 Article or 1 Chapter
- Cancel anytime
Buy Now
Price includes VAT (France)
eBook EUR 128.39 Price includes VAT (France)
Softcover Book EUR 168.79 Price includes VAT (France)
Hardcover Book EUR 168.79 Price includes VAT (France)
Tax calculation will be finalised at checkout
Purchases are for personal use only
Similar content being viewed by others
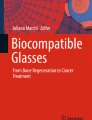
Bioactive Glasses: Advancing from Micro to Nano and Its Potential Application
Chapter © 2016
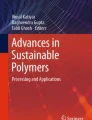
Bioactive Glasses: Prospects in Bone Tissue Engineering
Chapter © 2019
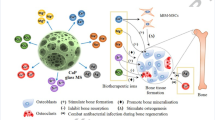
A better roadmap for designing novel bioactive glasses: effective approaches for the development of innovative revolutionary bioglasses for future biomedical applications
Article 02 December 2022
References
- Arvidson, K., et al.: Bone regeneration and stem cells. J. Cell. Mol. Chem. 15, 718–746 (2010) ArticleCASGoogle Scholar
- Williams, D.F.: On the mechanism of biocompatibility. Biomaterials 29, 2941–2953 (2008) ArticleCASGoogle Scholar
- Campana, V., et al.: Bone substitutes in orthopaedic surgery: from basic science to clinical practice. J. Mater. Sci. Mater. Med. 25, 2445–2461 (2014) ArticleCASGoogle Scholar
- Dimitriou, R., Mataliotakis, G.I., Angoules, A.G., Kanakaris, N.K., Giannoudis, P.V.: Complications following autologous bone graft harvesting from the iliac crest and using the RIA: a systematic review. Injury 42, S3–S15 (2011) ArticleGoogle Scholar
- Henkel, J., et al.: Bone regeneration based on tissue engineering conceptions—a 21st century perspective. Bone Res. 3, 216–248 (2013) ArticleCASGoogle Scholar
- Hench, L.L.: The story of Bioglass®. J. Mater. Sci. Mater. Med. 17, 967–978 (2006) ArticleCASGoogle Scholar
- Wilson, J., Pigott, G., Schoen, F., Hench, L.L.: Toxicology and biocompatibility of bioglasses. J. Biomed. Mater. Res. A 15, 805–817 (1981) ArticleCASGoogle Scholar
- Xynos, I., et al.: Bioglass® 45S5 stimulates osteoblast turnover and enhances bone formation in vitro: implications and applications for bone tissue engineering. Calcif. Tissue Int. 67, 321–329 (2000) ArticleCASGoogle Scholar
- Brink, M., Turunen, T., Happponen, R., Yli-Urpo, A.: Compositional dependence of bioactivity of glasses in the system Na2O-K2O-MgO-CaO-B2O3-P2O5-SiO2. J. Biomed. Mater. Res. 37, 114–121 (1997) ArticleCASGoogle Scholar
- Andersson, O.H., Liu, G., Karlsson, K.H., Juhanoja, J.: In vivo behavior of glasses in the SiO2–Na2O–CaO–P2O5–Al2O3–B2O3 system. J. Mater. Sci. Mater. Med. 1, 219–227 (1990) ArticleCASGoogle Scholar
- Knowles, J.C.: Phosphate based glasses for biomedical applications. J. Mater. Chem. 13, 2395–2401 (2003) ArticleCASGoogle Scholar
- Hench, L.L.: Bioactive ceramics. Ann. N. Y. Acad. Sci. 523, 54–71 (1988) ArticleCASGoogle Scholar
- Pantano, C.G., Clark, A.E., Hench, L.L.: Multilayer corrosion films on bioglass surfaces. J. Am. Ceram. Soc. 57, 412–413 (1974) ArticleCASGoogle Scholar
- Hench, L.L., Roki, N., Fenn, M.B.: Bioactive glasses: importance of structure and properties in bone regeneration. J. Mol. Struct. 1073, 24–30 (2014) ArticleCASGoogle Scholar
- Salinas, A.J., Vallet-Regí, M.: Bioactive ceramics: from bone grafts to tissue engineering. RSC Adv. 3, 11116–11131 (2013) ArticleCASGoogle Scholar
- Kokubo, T., Ito, S., Sakka, S., Yamamuro, T.: Formation of a high-strength bioactive glass-ceramic in the system MgO-CaO-SiO2-P2O5. J. Mater. Sci. 21, 536–540 (1986) ArticleCASGoogle Scholar
- Montazerian, M., Zanotto, E.D.: History and trends of bioactive glass-ceramics. J. Biomed. Mater. Res. A 104, 1231–1249 (2016) ArticleCASGoogle Scholar
- Hench, L.L.: Genetic design of bioactive glasses. J. Eur. Ceram. Soc. 29, 1257–1265 (2008) ArticleCASGoogle Scholar
- Jell, G., Stevens, M.M.: Gene activation by bioactive glasses. J. Mater. Sci. Mater. Med. 17, 997–1002 (2006) ArticleCASGoogle Scholar
- Kokubo, T., Takadama, H.: How useful is SBF in predicting in vivo bone bioactivity? Biomaterials 27, 2907–2915 (2006) ArticleCASGoogle Scholar
- Mizuno, M.: Implants for Surgery: In Vitro Evaluation for Apatite Forming Ability of Implant Materials (ISO ISO/DIS 23317:2014), last reviewed and confirmed in 2020 Google Scholar
- Bohner, M., Lemaitre, J.: Can bioactivity be tested in vitro with SBF solution? Biomaterials 30, 2175–2179 (2009) ArticleCASGoogle Scholar
- Macon, A.L.B., et al.: A unified in vitro evaluation for apatite-forming ability of bioactive glasses and their variants. J. Mater. Sci. Mater. Med. 26, 115 (2015) ArticleCASGoogle Scholar
- Mozafari, M., Banijamali, S., Baino, F., Kargozar, S., Hill, R.G.: Calcium carbonate: adored and ignored in bioactivity assessment. Acta Biomater. 91, 35–47 (2019) ArticleCASGoogle Scholar
- Kaur, G., Pickrell, G., Sriranganathan, N., Kumar, V., Homa, D.: Review and the state of the art: sol–gel and melt quenched bioactive glasses for tissue engineering. J. Biomed. Mater. Res. 104, 1248–1275 (2015) ArticleCASGoogle Scholar
- Hench, L.L., Splinter, R.J., Allen, W.C., Greenlee, T.K.: Bonding mechanisms at the interface of ceramic prosthetic materials. J. Biomed. Mater. Res. 5, 117–141 (1971) ArticleGoogle Scholar
- Vedel, E., Arstila, H., Ylanen, H., Hupa, L., Hupa, M.: Predicting physical and chemical properties of bioactive glasses from chemical composition. Part I. Viscosity characteristics. Glass Technol. Eur. J. Glass Sci. Technol. 49, 251–259 (2008) Google Scholar
- Li, R., Clarck, A.E., Hench, L.L.: An investigation of bioactive glass powders by sol-gel processing. J. Appl. Biomater. 2, 231–239 (1991) ArticleCASGoogle Scholar
- Graham, T.: On the properties of silicic acid and other analogous colloidal substances. J. Chem. Soc. 17, 318–327 (1864) ArticleGoogle Scholar
- Flory, P.J.: Principle of Polymer Chemistry. Cornell University Press, Ithaca and London (1953) Google Scholar
- Kakihana, M.: Invited review ‘‘sol–gel” preparation of high temperature superconducting oxides. J. Sol-Gel. Sci. Technol. 6, 7–55 (1996) ArticleCASGoogle Scholar
- Danks, A.E., Hall, S.R., Schnepp, Z.: The evolution of ‘sol–gel’ chemistry as a technique for materials synthesis. Mater. Horiz. 3, 91 (2016) ArticleCASGoogle Scholar
- Baino, F., Fiume, E., Miola, M., Verné, E.: Bioactive sol-gel glasses: processing, properties, and applications. Int. J. Appl. Ceram. Technol. 15, 841–860 (2018) ArticleCASGoogle Scholar
- Hench, L.L.: The sol-gel process. Chem. Rev. 90, 33–72 (1990) ArticleCASGoogle Scholar
- Kesmez, Ö., et al.: Effect of acid, water and alcohol ratios on sol-gel preparation of antireflective amorphous SiO2 coatings. J. Non-Cryst. Solids 357, 3130–3135 (2011) ArticleCASGoogle Scholar
- Arcos, D., Vallet-Regí, M.: Sol-gel silica-based biomaterials and bone tissue regeneration. Acta Biomater. 6, 2874–2888 (2010) ArticleCASGoogle Scholar
- Owens, G.J., et al.: Sol-gel based materials for biomedical applications. Prog. Mater Sci. 77, 1–79 (2016) ArticleCASGoogle Scholar
- Doadrio, J.C., et al.: Functionalization of mesoporous materials with long alkyl chains as a strategy for controlling drug delivery pattern. J. Mater. Chem. 16, 462–466 (2006) ArticleCASGoogle Scholar
- Wu, C., Zhang, Y., Zhou, Y., Fan, W., Xiao, Y.: A comparative study of mesoporous-glass/silk and nonmesoporous-glass/silk scaffolds: physio-chemistry and in vivo osteogenesis. Acta Biomater. 7, 2229–2236 (2011) ArticleCASGoogle Scholar
- Baino, F., Fiume, E.: 3D printing of hierarchical scaffolds based on mesoporous bioactive glasses (MBGs)-fundamentals and applications. Materials (Basel) 13, 1688 (2020) Google Scholar
- Lefebvre, L., Gremillardm, L., Chevalier, J., Zenati, R., Bernache-Assolant, D.: Sintering behaviour of 45S5 bioactive glass. Acta Biomater. 4, 1894–1903 (2008) ArticleCASGoogle Scholar
- Bellucci, D., et al.: Macroporous bioglass derived scaffolds for bone tissue regeneration. Ceram. Int. 37, 1575–1585 (2011) ArticleCASGoogle Scholar
- Arstilla, H., et al.: The sintering range of porous bioactive glasses. Glass Technol. 46, 138–141 (2005) Google Scholar
- Zhang, D., Vedel, E., Hupa, L., Aro, H.T.: Predicting physical and chemical properties of bioactive glasses from chemical composition. Part III. In vitro reactivity of glasses. Glass Technol. Eur. J. Glass Sci. Technol. 50, 1–8 (2009) Google Scholar
- Tulyaganov, D.U., et al.: Synthesis of glass-ceramics in the CaO–MgO–SiO2 system with B2O3, P2O5, Na2O and CaF2 additives. J. Eur. Ceram. Soc. 26, 1463–1471 (2006) ArticleCASGoogle Scholar
- Agathopoulos, S., Tulyaganov, D.U., Ventura, J.M.G., Kannan, S., Saranti, A., Karakassides, M.A.: Structural analysis and devitrification of glasses based on the CaO–MgO–SiO2 system with B2O3, P2O5, Na2O and CaF2 additives. J. Non-Cryst. Solids 352, 322–328 (2006) Google Scholar
- Kansal, I., Tulyaganov, D.U., Goel, A., Pascual, M.J., Ferreira, J.M.F.: Structural analysis and thermal behaviour of diopside-fluorapatite-wollastonite-based glasses and glass-ceramics. Acta Biomater. 6, 4380–4388 (2010) ArticleCASGoogle Scholar
- Filho, O.P., Latorre, G.P., Hench, L.L.: Effect of crystallization on apatite-layer formation of bioactive glass 45S5. J. Biomed. Mater. Res. 30, 509–514 (1996) ArticleGoogle Scholar
- Peitl, O., Dutra, E., Hench, L.L.: Highly bioactive P 2 O 5 ± Na 2 O ± CaO ± SiO 2 glass-ceramics. J. Non-Cryst. Solids 292, 115–126 (2001) ArticleCASGoogle Scholar
- Arstila, H., Hupa, L., Karlson, K., Hupa, M.: In vitro bioactivity of partially crystallised glasses. Glass Technol. Eur. J. Glass Sci. Technol. A 48, 196–199 (2007) CASGoogle Scholar
- De Castro Juraski, A., Dorion Rodas, A.C., Elsayed, H., Bernardo, E., Oliveira Soares, V., Daguano, J.: The in vitro bioactivity, degradation, and cytotoxicity of polymer-derived wollas-tonite-diopside glass-ceramics. Materials 10, 425 (2017) ArticleCASGoogle Scholar
- Fu, Q., Rahaman, M.N., Bal, B.S., Brown, R.F., Day, D.E.: Mechanical and in vitro performance of 13–93 bioactive glass scaffolds prepared by a polymer foam replication technique. Acta Biomater. 4, 1854–1864 (2008) ArticleCASGoogle Scholar
- Liu, X., Rahaman, M.N., Hilmas, G.E., Bal, B.S.: Mechanical properties of bioactive glass (13–93) scaffolds fabricated by robotic deposition for structural bone repair. Acta Biomater. 9, 7025–7034 (2013) ArticleCASGoogle Scholar
- Massera, J., Fagerlund, S., Hupa, L., Hupa, M.: Crystallization mechanism of the bioactive glasses, 45S5 and S53P4. J. Am. Ceram. Soc. 95, 603–613 (2012) ArticleCASGoogle Scholar
- Baino, F., Fiume, E.: Quantifying the effect of particle size on the crystallization of 45S5 bioactive glass. Mater. Lett. 224, 54–58 (2018) ArticleCASGoogle Scholar
- Fiume, E., Migneco, C., Verné, E., Baino, F.: Comparison between bioactive sol-gel and melt-derived glasses/glass-ceramics based on the multicomponent SiO2–P2O5–CaO–MgO–Na2O–K2O system. Materials (Basel) 13, 540 (2020) Google Scholar
- Montazerian, M., Zanotto, E.D.: Bioactive and inert dental glass-ceramics. J. Biomed. Mater. Res. Part A 105, 619–639 (2017) ArticleCASGoogle Scholar
- Nedelec, J.-M., et al.: Materials doping through sol–gel chemistry: a little something can make a big difference. J. Sol-Gel. Sci. Technol. 46, 259–271 (2008) ArticleCASGoogle Scholar
- Rabiee, S.M., Nazparvar, N., Azizian, M., Vashaee, D., Tayebi, L.: Effect of ion substitution on properties of bioactive glasses: a review. Ceram. Int. 41, 7241–7251 (2015) Google Scholar
- Wu, C., Chang, J.: Multifunctional mesoporous bioactive glasses for effective delivery of therapeutic ions and drug/growth factors. J. Control. Release 193, 282–295 (2014) ArticleCASGoogle Scholar
- Krajewski, A., Ravaglioli, A., Fabbri, B., Azzoni, C.B.: Doping influence on the interaction between a bioactive glass and a simulated physiological solution: Chemical and EPR tests. J. Mater. Sci. 22, 1228–1234 (1987) ArticleCASGoogle Scholar
- Abou Neel, E.A., Knowles, J.C.: Physical and biocompatibility studies of novel titanium dioxide doped phosphate-based glasses for bone tissue engineering applications. J. Mater. Sci. Mater. Med. 19, 377–386 (2008) Google Scholar
- Yin, H., et al.: Fabrication and characterization of strontium-doped borate-based bioactive glass scaffolds for bone tissue engineering. J. Alloy. Compd. 743, 564–569 (2018) ArticleCASGoogle Scholar
- Beattie, J.H., Avenell, A.: Trace element nutrition and bone metabolism. Nutr. Res. Rev. 5, 167–188 (1992) ArticleCASGoogle Scholar
- Fiume, E., Barberi, J., Verné, E., Baino, F.: Bioactive glasses: from parent 45S5 composition to scaffold-assisted tissue-healing therapies. J. Funct. Biomater. 9, 24 (2018) ArticleCASGoogle Scholar
- Hutmacher, D.W.: Scaffolds in tissue engineering bone and cartilage. Biomaterials 21, 2529–2543 (2000) ArticleCASGoogle Scholar
- Karageorgiou, V., Kaplan, D.: Porosity of 3D biomaterial scaffolds and osteogenesis. Biomaterials 26, 5474–5491 (2005) ArticleCASGoogle Scholar
- Chappard, D., Baslé, M.F., Legrand, E., Audran, M.: New laboratory tools in the assessment of bone quality. Osteoporos. Int. 22, 2225–2240 (2011) ArticleCASGoogle Scholar
- Gerhardt, L.-C., Boccaccini, A.R.: Bioactive glass and glass-ceramic scaffolds for bone tissue engineering. Materials (Basel) 3, 3867–3910 (2010) ArticleCASGoogle Scholar
- Fu, Q., Saiz, E., Rahaman, M.N., Tomsia, A.P.: Bioactive glass scaffolds for bone tissue engineering: state of the art and future perspectives. Mater. Sci. Eng., C 31, 1245–1256 (2011) ArticleCASGoogle Scholar
- Baino, F., Vitale-Brovarone, C.: Three-dimensional glass-derived scaffolds for bone tissue engineering: current trends and forecasts for the future. J. Biomed. Mater. Res. Part A 97, 514–535 (2011) ArticleCASGoogle Scholar
- Baino, F., et al.: Processing methods for making porous bioactive glass-based scaffolds—a state-of-the-art review. Int. J. Appl. Ceram. Technol. 16, 1762–1796 (2019) ArticleCASGoogle Scholar
- Xynos, I.D., Edgar, A.J., Buttery, L.D.K., Hench, L.L., Polak, J.M.: Gene-expression profiling of human osteoblasts following treatment with the ionic products of Bioglass® 45S5 dissolution. J. Biomed. Mater. Res. 55, 151–157 (2001) ArticleCASGoogle Scholar
- Yuan, H., de Bruijn, J.D., Zhang, X., Van Blitterswijk, C.A., De Groot, K.: Bone induction by porous glass ceramic made from Bioglass® (45S5). J. Biomed. Mater. Res. 58, 270–276 (2002) ArticleGoogle Scholar
- Sepulveda, P., Jones, J.R., Hench, L.L.: Bioactive sol–gel foams for tissue repair. J. Biomed. Mater. Res. 59, 340–348 (2002) ArticleCASGoogle Scholar
- Jones, J.R., Lee, P.D., Hench, L.L.: Hierarchical porous materials for tissue engineering. Phil. Trans. R. Soc. A 364, 263–281 (2006) ArticleCASGoogle Scholar
- Baino, F., Fiume, E.: Elastic mechanical properties of 45S5-based bioactive glass-ceramic scaffolds. Materials (Basel) 12 (2019) Google Scholar
- Baino, F., Pons, E.: Modelling the relationship between tensile strength and porosity in bioceramic scaffolds. Int. J. Appl. Ceram. Technol. 16, 1823–1829 (2019) ArticleCASGoogle Scholar
- Kargozar, S., et al.: Synergistic combination of bioactive glasses and polymers for enhanced bone tissue regeneration. Mater Today Proc. 5, 15532–15539 (2018) ArticleCASGoogle Scholar
- Bretcanu, O., et al.: Biodegradable polymer coated 45S5 bioglass derived glass-ceramic scaffolds for bone tissue engineering. Glass Technol. Eur. J. Glass Sci. Technol. A 48, 227–234 (2007) CASGoogle Scholar
- Rehorek, L., et al.: Response of 45S5 bioglass_foams to tensile loading. Ceram. Int. 39, 8015–8020 (2013) ArticleCASGoogle Scholar
- Jones, J.R.: Review of bioactive glass: from hench to hybrids. Acta Biomater. 9, 4457–4486 (2013) ArticleCASGoogle Scholar
- Baino, F., Novajra, G., Vitale-Brovarone, C.: Bioceramics and scaffolds: a winning combination for tissue engineering. Front. Bioeng. Biotechnol. 3, 1–17 (2015) ArticleGoogle Scholar
- Wu, Z.Y., et al.: Melt-derived bioactive glass scaffolds produced by a gel-cast foaming technique. Acta Biomater. 7, 1807–1816 (2011) ArticleCASGoogle Scholar
- Poologasundarampillai, G., Lee, P.D., Lam, C., Kourkouta, A.M., Jones, J.R.: Compressive strength of bioactive sol-gel glass foam scaffolds. Int. J. Appl. Glas. Sci. 9, 1–9 (2016) Google Scholar
- Jones, J.R., Ehrenfried, L.M., Hench, L.L.: Optimizing bioactive glass scaffolds for bone tissue engineering. Biomaterials 27, 964–973 (2006) ArticleCASGoogle Scholar
- Jones, J.R., Ehrenfried, L.M., Saravanapavan, P., Hench, L.L.: Controlling ion release from bioactive glass foam scaffolds with antibacterial properties. J. Mater. Sci. Mater. Med. 17, 989–996 (2006) ArticleCASGoogle Scholar
- Baino, F., et al.: Fe-doped bioactive glass-derived scaffolds produced by sol-gel foaming. Mater. Lett. 235, 207–211 (2019) ArticleCASGoogle Scholar
- Navarro, M., et al.: New macroporous calcium phosphate glass ceramic for guided bone regeneration. Biomaterials 25, 4233–4241 (2004) ArticleCASGoogle Scholar
- Fiume, E., et al.: Comprehensive assessment of bioactive glass and glass-ceramic scaffold permeability: experimental measurements by pressure wave drop, modelling and computed tomography-based analysis. Acta Biomater. 119, 405–418 (2021) ArticleCASGoogle Scholar
- Boccardi, E., et al.: Characterisation of Bioglass based foams developed via replication of natural marine sponges. Adv. Appl. Ceram. 114, S56–S62 (2015) ArticleCASGoogle Scholar
- Fiume, E., Serino, G., Bignardi, C., Verné, E., Baino, F.: Bread-derived bioactive porous scaffolds: an innovative and sustainable approach to bone tissue engineering. Molecules 24, 2954 (2019) ArticleCASGoogle Scholar
- Vitale-Brovarone, C., Vernè, E., Bosetti, M., Appendino, P., Cannas, M.: Microstructural and in vitro characterization of SiO2-Na2O-CaO-MgO glass-ceramic bioactive scaffolds for bone substitutes. J. Mater. Sci. Mater. Med. 16, 909–917 (2005) ArticleCASGoogle Scholar
- Baino, F., Verné, E., Vitale-Brovarone, C.: 3-D high strength glass-ceramic scaffolds containing fluoroapatite for load-bearing bone portions replacement. Mater. Sci. Eng., C 29, 2055–2062 (2009) ArticleCASGoogle Scholar
- Liang, W., et al.: Bioactive borate glass scaffold for Bone tissue engineering. J. Non-Cryst. Solids 354, 1690–1696 (2008) ArticleCASGoogle Scholar
- Fiume, E., Tulyaganov, D., Ubertalli, G., Verné, E., Baino, F.: Dolomite-foamed bioactive silicate scaffolds for bone tissue repair. Materials 13, 628 (2020) ArticleCASGoogle Scholar
- Minaberry, Y., Jobbágy, M.: Macroporous bioglass scaffolds prepared by coupling sol-gel with freeze drying. Chem. Mater. 23, 2327–2332 (2011) ArticleCASGoogle Scholar
- Maquet, V., Boccaccini, A.R., Pravata, L., Notingher, I., Jérôme, R.: Porous poly(α‐hydroxyacid)/bioglass® composite scaffolds for bone tissue engineering. I: preparation and in vitro characterization. Biomaterials 25, 4185–4194 (2004) Google Scholar
- Gmeiner, R., et al.: Additive manufacturing of bioactive glasses and silicate bioceramics. J. Ceram. Sci. Technol. 6, 75–86 (2015) Google Scholar
- Liu, J., Hu, H., Li, P., Shuai, C., Peng, S.: Fabrication and characterization of porous 45S5 glass scaffolds via direct selective laser sintering. Mater. Manuf. Processes 28, 610–615 (2013) CASGoogle Scholar
- Tesavibul, P., et al.: Processing of 45S5 bioglass® by lithography-based additive manufacturing. Mater. Lett. 41, 81–84 (2012) ArticleCASGoogle Scholar
- Bose, S., Vahabzadeh, S., Bandyopadhyay, A.: Bone tissue engineering using 3D printing. Mater. Today 16, 496–504 (2013) ArticleCASGoogle Scholar
- Barberi, J., et al.: Robocasting of SiO2-based bioactive glass scaffolds with porosity gradient for bone regeneration and potential load-bearing applications. Materials (Basel) 12 (2019) Google Scholar
- Yun, H.S., Kim, S.E., Park, E.K.: Bioactive glass-poly(epsilon-caprolactone) composite scaffolds with 3 dimensionally hierarchical pore networks. Mater. Sci. Eng., C 31, 198–205 (2011) ArticleCASGoogle Scholar
- McEntire, B., Bal, B., Rahaman, M., Chevalier, J., Pezzotti, G.: Ceramics and ceramic coatings in orthopaedics. J. Eur. Ceram. Soc. 35, 4327–4369 (2015) ArticleCASGoogle Scholar
- Sun, L., Berndt, C.C., Gross, K.A., Kucuk, A.: Material fundamentals and clinical performance of plasma-sprayed hydroxyapatite coatings: a review. J. Biomed. Mater. Res. Part A 58, 570–592 (2001) ArticleCASGoogle Scholar
- Sola, A., Bellucci, D., Cannillo, V., Cattini, A.: Bioactive glass coatings: a review. Surf. Eng. 27, 560–572 (2011) ArticleCASGoogle Scholar
- Lopez-Esteban, S., et al.: Bioactive glass coatings for orthopedic metallic implants. J. Eur. Ceram. Soc. 23, 2921–2930 (2003) ArticleCASGoogle Scholar
- Verne, E., Brovarone, C.V., Moisescu, C.: Glazing of alumina by a fluoroapatite-containing glass-ceramic. J. Mater. Sci. 40, 1209–1215 (2005) Google Scholar
- Bosetti, M., et al.: Fluoroapatite glass-ceramic coating on alumina: surface behaviour with biological fluids. J. Biomed. Mater. Res. 66A, 615–621 (2003) ArticleCASGoogle Scholar
- Verne, E., Fernandez-Valles, C., Brovarone, C.V., Spriano, S., Moisescu, C.: Double-layer glass-ceramic coatings on Ti6Al4V for dental implants. J. Eur. Ceram. Soc. 24, 2699–2705 (2004) Google Scholar
- Verné, E., Bona, E., Angelini, E., Rosalbino, F., Appendino, P.: Correlation between microstructure and properties of biocomposite coatings. J. Eur. Ceram. Soc. 22, 2315–2323 (2002) ArticleGoogle Scholar
- Vernè, E., et al.: Fluoroapatite glass-ceramic coatings on alumina: structural, mechanical and biological characterisation. Biomaterials 23, 3395–3403 (2002) ArticleGoogle Scholar
- Ferraris, M., et al.: Coatings on zirconia for medical applications. Biomaterials 21, 765–773 (2000) ArticleCASGoogle Scholar
- Verné, E., Ferraris, M., Jana, C., Paracchini, L.: Bioverit®I base glass/Ti par-ticulate biocomposite: “in situ” vacuum plasma spray deposition. J. Eur. Ceram. Soc. 20, 473–479 (2000) ArticleGoogle Scholar
- Verné, E., et al.: Sintering and plasma spray deposition of bioactive glass-matrix composites for biomedical applications. J. Eur. Ceramic Soc. 18, 363–372 (1998) Google Scholar
- Alonso-Barrio, J.A., et al.: Bioglass-coated femoral stem. J. Bone Joint Surg. 86-B, 138 (2004) Google Scholar
- Gomez-Vega, J.M., et al.: Novel bioactive functionally graded coatings on Ti6Al4V. Adv. Mater. 12, 894–898 (2000) ArticleCASGoogle Scholar
- Boccaccini, A.R., Keim, S., Ma, R., Li, Y., Zhitomirsky, I.: Electrophoretic deposition of biomaterials. J. Royal Soc. Interf. 7 (2010) Google Scholar
- Baino, F., et al.: Novel antibacterial ocular prostheses: proof of concept and physico-chemical characterization. Mater. Sci. Eng., C 60, 467–474 (2016) ArticleCASGoogle Scholar
- Moskalewicz, T., Seuss, S., Boccaccini, A.R.: Microstructure and properties of composite poly-etheretherketone/Bioglass® coatings deposited on Ti-6Al-7Nb alloy for medical applications. Appl. Surf. Sci. 273, 62–67 (2013) ArticleCASGoogle Scholar
- Seuss, S., Heinloth, M., Boccaccini, A.R.: Development of bioactive composite coatings based on combination of PEEK, bioactive glass and ag nanoparticles with antimicrobial properties. Surf. Coat. Technol. 301, 100–105 (2016) ArticleCASGoogle Scholar
- Baino, F., Verné, E.: Glass-based coatings on biomedical implants: a state-of-the-art review. Biomed. Glass. 3, 1–17 (2017) ArticleGoogle Scholar
- Ferraris, M., et al.: Chemical, mechanical, and antibacterial properties of silver nanocluster–silica composite coatings obtained by sputtering. Adv. Eng. Mater. 12, B276-282 (2010) ArticleCASGoogle Scholar
- Ferraris, M., Chiaretta, D., Fokine, M., Miola, M., Verné, E.: Pellicole antibatteriche ottenute da sputtering e procedimento per conferire proprietà antibatteriche ad un substrato, TO2008A000098 (2008) Google Scholar
- Ferraris, M., Balagna, C., Perero, S.: Method for the application of an antiviral coating to a substrate and relative coating, WO2019/082001 (2019) Google Scholar
- Muzio, G., et al.: Biocompatibility versus peritoneal mesothelial cells of polypropylene prostheses for hernia repair, coated with a thin silica/silver layer. J. Biomed. Mater. Res. B Appl. Biomater. 105, 1586–1593 (2017) ArticleCASGoogle Scholar
- Irfan, M., et al.: Antimicrobial functionalization of cotton fabric with silver nanoclusters/silica composite coating via RF co-sputtering technique. Cellulose 24, 2331–2345 (2017) ArticleCASGoogle Scholar
- Balagna, C., et al.: Characterization of antibacterial silver nanocluster/silica composite coating on high performance Kevlar® textile. Surf. Coat. Technol. 321, 438–447 (2017) ArticleCASGoogle Scholar
- Miola, M., et al.: Silver nanocluster-silica composite antibacterial coatings for materials to be used in mobile telephones. Appl. Surf. Sci. 313, 107–115 (2014) ArticleCASGoogle Scholar
- Balagna, C., et al.: Antipathogen nanostructured coating for air filters. Appl. Surf. Sci. 508, 145283 (2020) Google Scholar
- Balagna, C., et al.: Antibacterial coating on polymer for space application. Mater. Chem. Phys. 135, 714–722 (2012) ArticleCASGoogle Scholar
- Balagna, C., Francese, R., Perero, S., Lembo, D., Ferraris, M.: Nanostructured composite coating endowed with antiviral activity against human respiratory viruses deposited on fibre-based air filters. Surf. Coat. Technol. 409, 126873 (2021) Google Scholar
- Balagna, C., Perero, S., Percivalle, E., Vecchio Nepita, E., Ferraris, M.: Virucidal effect against coronavirus SARS-CoV-2 of a silver nanocluster/silica composite sputtered coating. Open Ceram. 1, 100006 (2020) Google Scholar
- Verné, E., Vitale-Brovarone, C., Robiglio, I., Baino, F.: Single-Piece Ceramic Prosthesis Elements, EP2152328A2 (2008) Google Scholar
- Baino, F., Vitale-Brovarone, C.: Feasibility of glass-ceramic coatings on alumina prosthetic implants by airbrush spraying method. Ceram. Int. 41, 2150–2159 (2015) ArticleCASGoogle Scholar
- Baino, F., et al.: Novel full-ceramic monoblock acetabular cup with a bioactive tra-becular coating: design. Fabr. Charact. Ceram. Int. 42, 6833–6845 (2016) ArticleCASGoogle Scholar
- Baino, F., Vitale-Brovarone, C.: Trabecular coating on curved alumina substrates using a novel bio-active and strong glass-ceramic. Biomed. Glass. 1, 31–40 (2015) Google Scholar
- Baino, F., Montealegre, M.A., Orlygsson, G., Novajra, G., Vitale-Brovarone, C.: Bioactive glass coatings fabricated by laser cladding on ceramic acetabular cups: a proof-of-concept study. J. Mater. Sci. 52, 9115–9128 (2017) ArticleCASGoogle Scholar
- Cevc, G., Blume, G.: Hydrocortisone and dexamethasone in very deformable drug carriers have increased biological potency, prolonged effect, and reduced ther-apeutic dosage. Biochem. Biophys. Acta. 1663, 61–73 (2004) ArticleCASGoogle Scholar
- Hum, J., Boccaccini, A.R.: Bioactive glasses as carriers for bioactive molecules and therapeutic drugs: a review. J. Mater. Sci. Mater. Med. 23, 2317–2333 (2012) ArticleCASGoogle Scholar
- Arcos, D., Vallet-Regí, M.: Bioceramics for drug delivery. Acta Mater. 61, 890–911 (2013) ArticleCASGoogle Scholar
- Yan, X., Yu, C., Zhou, X., Tang, J., Zhao, D.: Highly ordered mesoporous bioactive glasses with superior in vitro bone-forming bioactivities. Angewandte Chemie Int. Ed. Engl. 43, 5980–5984 (2004) ArticleCASGoogle Scholar
- Migneco, C., Fiume, E., Verné, E., Baino, F.: A Guided walk through the world of mesoporous bioactive glasses (MBGs): fundamentals, processing, and applications. Nanomaterials 10, 2571 (2020) ArticleCASGoogle Scholar
- Izquierdo-Barba, I., Vallet-Regi, M.: Mesoporous bioactive glasses: relevance of their porous structure compared to that of classical bioglasses. Biomed. Glass. 1, 140–150 (2015) Google Scholar
- Wu, C., et al.: Bioactive mesopore-glass microspheres with controllable protein-delivery properties by biomimetic surface modification. J. Biomed. Mater. Res. A 95, 476–485 (2010) ArticleCASGoogle Scholar
- Zhao, L.Z., et al.: Mesoporous bioactive glasses for controlled drug release. Micropor. Mesop. Mater. 109, 210–215 (2008) ArticleCASGoogle Scholar
- Xia, W., Chang, J.: Well-ordered mesoporous bioactive glasses (MBG): a promising bioac-tive drug delivery system. J. Control. Release 110, 522–530 (2006) ArticleCASGoogle Scholar
- Zhao, Y.F., Loo, S.C., Chen, Y.Z., Boey, F.Y., Ma, J.: In situ SAXRD study of sol-gel induced well-ordered mesoporous bioglasses for drug delivery. J. Biomed. Mater. Res. A 85, 1032–1042 (2008) ArticleCASGoogle Scholar
- López-Noriega, A., et al.: Ordered mesoporous bioactive glasses for bone tissue regeneration. Chem. Mater. 18, 3137–3144 (2006) ArticleCASGoogle Scholar
- Baino, F., Fiorilli, S., Vitale-Brovarone, C.: Bioactive glass-based materials with hierarchical porosity for medical applications: review of recent advances. Acta Biomater. 42, 18–32 (2016) ArticleCASGoogle Scholar
- Kargozar, S., Montazerian, M., Hamzehlou, S., Kim, H.W., Baino, F.: Mesoporous bioactive glasses: promising platforms for antibacterial strategies. Acta Biomater. 81, 1–19 (2018) ArticleCASGoogle Scholar
- Ahmed, A.A., Ali, A.A., Mahmoud, D.A.R., El-Fiqi, A.M.: Preparation and characterization of antibacterial P2O5–CaO–Na2O–Ag2O glasses. J. Biomed. Mater. Res. Part A 98A, 132–142 (2011) ArticleCASGoogle Scholar
- Bellantone, M., Coleman, N.J., Hench, L.L.: A novel sol-gel derived bioactive glass featuring antibacterial properties. Key Eng. Mater. 192–195, 597–600 (2001) Google Scholar
- Mulligan, A.M., Wilson, M., Knowles, J.C.: The effect of increasing copper 770 content in phosphate-based glasses on biofilms of Streptococcus sanguis, 771. Biomaterials 24, 1797–1807 (2003) ArticleCASGoogle Scholar
- Schuhladen, K., et al.: Cu, Zn doped borate bioactive glasses: antibacterial efficacy and dose-dependent in vitro modulation of murine dendritic cells. Biomater. Sci. 8, 2143 (2020) ArticleCASGoogle Scholar
- Vernè, E., et al.: Surface silver-doping of biocompatible glass to induce antibacterial properties. Part I: massive glass. J. Mater. Sci. Mater. Med. 20, 2935–2942 (2009) Google Scholar
- Miola, M., Vernè, E.: Bioactive and antibacterial glass powders doped with copper by ion-exchange in aqueous solutions. Materials 9, 405 (2016) ArticleCASGoogle Scholar
- Di Nunzio, S., et al.: Silver containing bioactive glasses prepared by molten salt ion-exchange. J. Eur. Ceram. Soc. 24, 2935–2942 (2004) ArticleCASGoogle Scholar
- Bellantone, M., Williams, H.D., Hench, L.L.: Broad-spectrum bactericidal activity of Ag (2)O-doped bioactive glass. Antimicrob. Agents Chemother. 46, 1940–1945 (2002) ArticleCASGoogle Scholar
- Diba, M., Boccaccini, A.R.: Silver‐containing bioactive glasses for tissue engineering applications. In: Baltzer, N., Copponnex, T. (eds.) Precious Metals for Biomedical Applications, pp. 177–211. Woodhead Publishing (2014) Google Scholar
- Neel, E.A., Ahmed, I., Pratten, J., Nazhat, S.N., Knowles, J.C.: Characterisation of antibacterial copper releasing degradable phosphate glass fibres. Biomaterials 26, 2247–2254 (2005) ArticleCASGoogle Scholar
- Baghbani, F., Moztarzadeh, F., Hajibaki, L., Mozafari, M.: Synthesis, characterization and evaluation of bioactivity and antibacterial activity of quinary glass system (SiO2–CaO–P2O5–MgO–ZnO): in vitro study. Bull. Mater. Sci. 36, 1339–1346 (2013) ArticleCASGoogle Scholar
- Valappil, S.P., et al.: Role of gallium and silver from phosphate‐based glasses on in vitro dual species oral biofilm models of Porphyromonas gingivalis and Streptococcus gordonii. Acta Biomater. 8, 1957–1965 (2012) Google Scholar
- Sakthi, P.S., et al.: In vitro bioactivity and antibacterial properties of bismuth oxide modified bioactive glasses. J. Mater. Res. 33, 178–190 (2018) ArticleCASGoogle Scholar
- Liu, L., Pushalkar, S., Saxena, D., LeGeros, R.Z., Zhang, Y.: Antibacterial property expressed by a novel calcium phosphate glass. J. Biomed. Mater. Res. B Appl. Biomater. 102, 423–429 (2014) ArticleCASGoogle Scholar
- Marta, M., et al.: Tellurium: a new active element for innovative multifunctional bioactive glasses. Mater. Sci. Eng. C 123, 111957 (2021) Google Scholar
- Gruian, K.M.C., et al.: Addressing the optimal silver content in bioactive glass systems in terms of BSA adsorption. J. Mater. Chem. B 2, 5799–5808 (2014) ArticleCASGoogle Scholar
- Verné, E., et al.: Synthesis and characterisation of bioactive and antibacterial glass–ceramic part 1—microstructure, properties and biological behaviour. Adv. Appl. Ceram. 107, 234–244 (2008) ArticleCASGoogle Scholar
- Vitale-Brovarone, C., Miola, M., Balagna, C., Verné, E.: 3D-glass–ceramic scaffolds with antibacterial properties for bone grafting. Chem. Eng. J. 137, 129–136 (2008) ArticleCASGoogle Scholar
- Gholipourmalekabadi, M., et al.: Detection and qualification of optimum antibacterial and cytotoxic activities of silver-doped bioactive glasses. ET Nanobiotechnol. 9, 209–214 (2015) ArticleGoogle Scholar
- Wilkinson, H.N., Iveson, S., Catherall, P., Hardman, M.J.: A novel silver bioactive glass elicits antimicrobial efficacy against Pseudomonas aeruginosa and Staphylococcus aureus in an ex vivo skin wound biofilm model. Front. Microbiol. 9, 1450 (2018) ArticleGoogle Scholar
- Miola, M., et al.: Antibiotic-free composite bone cements with antibacterial and bioactive proper-ties. A preliminary study. Mater. Sci. Eng. C 43, 65–75 (2014) Google Scholar
- Miola, M., Verné, E., Vitale-Brovarone, C., Baino, F.: Antibacterial bioglass-derived scaffolds: innovative synthesis approach and characterization. Int. J. Appl. Glas. Sci. 7, 238–247 (2016) ArticleCASGoogle Scholar
- Miola, M., Fucale, G., Maina, G., Verné, E.: Composites bone cements with different viscosities loaded with a bioactive and antibacterial glass. J. Mater. Sci. 52, 5133–5146 (2017) ArticleCASGoogle Scholar
- Miola, M., Fucale, G., Maina, G., Verné, E.: Antibacterial and bioactive composite bone cements containing surface silver-doped glass particles. Biomed. Mater. 10, 055041 (2015) Google Scholar
- Hosny, A.E.-D.M., Rasmy, S.A., Aboul-Magd, D.S., Kashef, M.T., El-Bazza, Z.E.: The increasing threat of silver-resistance in clinical isolates from wounds and burns. Inf. Drug Resist. 12, 1985–2001 (2019) Google Scholar
- Aina, V., et al.: Influence of the chemical composition on nature and activity of the surface layer of Zn-substituted sol-gel (Bioactive) glasses. J. Phys. Chem. C 115, 2196–2210 (2011) ArticleCASGoogle Scholar
- Raffi, M., et al.: Investigations into the antibacterial behavior of copper nanoparticles against Escherichia coli. Ann. Microbiol. 60, 75–80 (2010) ArticleCASGoogle Scholar
- Goh, Y.F., et al.: Bioactive glass: an in-vitro comparative study of doping with nanoscale copper and silver particles. Appl. Glass Sci. 5, 255–266 (2014) ArticleCASGoogle Scholar
- Srivastava, A.K., Pyare, R.: Characterization of CuO substituted 45S5 bioactive glasses and glass-ceramics. Int. J. Sci. Technol. Res. 1, 28–41 (2012) Google Scholar
- Miola, M., et al.: Copper-doped bioactive glass as filler for PMMA-based bone cements: morphological, mechanical, reactivity, and preliminary antibacterial characterization. Materials (Basel) 11, 961 (2018) Google Scholar
- Esteban-Tejeda, L., et al.: Calcium and zinc containing bactericidal glass coatings for biomedical metallic substrates. Int. J. Mol. Sci. 15, 13030–13044 (2014) ArticleCASGoogle Scholar
- Balasubramanian, P., Strobel, L.A., Kneser, U., Boccaccini, A.R.: Zinc-containing bioactive glasses for bone regeneration, dental and orthopedic applications. Biomed. Glass. 1, 51–69 (2015) Google Scholar
- Shahrbabak, M.S.N., Sharifianjazi, F., Rahban, D., Salimi, A.: A comparative investigation on bioactivity and antibacterial properties of sol-gel derived 58S bioactive glass substituted by Ag and Zn. Silicon 11, 2741–2751 (2019) ArticleCASGoogle Scholar
- Grandi, S., et al.: Bone reconstruction: Au nanocomposite bioglasses with antibacterial properties. Int. J. Artif. Organs 34, 920–928 (2011) ArticleCASGoogle Scholar
- Vulpoi, A., et al.: Bioactivity and protein attachment onto bioactive glasses containing silver nanoparticles. J. Biomed. Mater. Res. A 100, 1179–1186 (2012) ArticleCASGoogle Scholar
- Ferraris, S., et al.: In situ reduction of antibacterial silver ions to metallic silver nanoparticles on bioactive glasses functionalized with polyphenols. Appl. Surf. Sci. 396, 461–470 (2017) ArticleCASGoogle Scholar
- Miola, M., Bertone, E., Vernè, E.: In situ chemical and physical reduction of copper on bioactive glass surface. Appl. Surf. Sci. 495, 143559 (2019) Google Scholar
- Hamad, A., Khashan, K.S., Hadi, A.: Silver nanoparticles and silver ions as potential antibacterial agents. J. Inorg. Organomet. Polym. 30, 4811–4828 (2020) ArticleCASGoogle Scholar
- Miola, M., Vitale-Brovarone, C., Mattu, C., Verné, E.: Antibiotic loading on bioactive glasses and glass-ceramics: an approach to surface modification. J. Biomater. Appl. 28, 308–319 (2012) ArticleCASGoogle Scholar
- Arcos, D., Lopez-Noriega, A., Ruiz-Hernandez, E., Terasaki, O., Val-let-Regi, M.: Ordered mesoporous microspheres for bone grafting and drug delivery. Chem. Mater. 21, 1000–1009 (2009) ArticleCASGoogle Scholar
- Anand, A., Das, P., Nandi, S.K., Kundua, B.: Development of antibiotic loaded mesoporous bioactive glass and its drug release kinetics. Ceram. Int. 46, 5477–5483 (2020) ArticleCASGoogle Scholar
- Cheng, T., Qu, H., Zhang, G., Zhang, X.: Osteogenic and antibacterial properties of vancomycin-laden mesoporous bio-glass/PLGA composite scaffolds for bone regeneration in infected bone defects. Artif. Cells, Nanomed. Biotechnol. 46, 1935–1947 (2018) CASGoogle Scholar
- Bortuzzo, K.Z.J.A., et al.: Bio-templated bioactive glass particles with hierarchical macro–nano porous structure and drug delivery capability. Colloids Surf. B Biointerf. 135, 825–832 (2015) ArticleCASGoogle Scholar
- Zhang, X., et al.: Teicoplanin-loaded borate bioactive glass implants for treating chronic bone infection in a rabbit tibia osteomyelitis model. Biomaterials 31, 5865–5874 (2010) ArticleCASGoogle Scholar
- Drago, L., Toscano, M., Bottagisio, M.: Recent evidence on bioactive glass antimicrobial and antibiofilm activity: a mini-review. Materials 11, 326 (2018) ArticleCASGoogle Scholar
- Gorustovich, J., Rivadeneira, A.: Bioactive glasses as delivery systems for antimicrobial agents. J. Appl. Microbiol. 122, 1424–1437 (2017) ArticleGoogle Scholar
- Hu, S., Chang, J., Liu, M., Ning, C.: Study on antibacterial effect of 45S5 Bioglass. J. Mater. Sci. Mater. Med. 20, 281–286 (2009) ArticleCASGoogle Scholar
- Rivadeneira, J., Audisio, C.M., Boccaccini, A.R., Gorustovich, A.A.: In vitro antistaphylococcal effects of a novel 45S5 bioglass/agar-gelatin biocomposite films. J. Appl. Microbiol. 115, 604–612 (2013) ArticleCASGoogle Scholar
- Drago, L., et al.: In vitro antibiofilm activity of bioactive glass S53P4. Future Microbiol. 9, 593–601 (2014) ArticleCASGoogle Scholar
- Saima Begum, J.W.E., Worthingtonand, T., Martin, R.A.: The influence of pH and fluid dynamics on the antibacterial efficacy of 45S5 Bio-glass. Biomed. Mater. 11, 015006 (2016) Google Scholar
- Xie, Z.P., et al.: Failure of particulate bioglass to prevent experimental staphylococcal infection of open tibial fractures. J. Antimicrob. Chemother. 62, 1162–1163 (2008) ArticleCASGoogle Scholar
- Verné, E., Ferraris, S.: Surface functionalization of bioactive glasses: reactive groups, biomolecules and drugs on bioactive surfaces for smart and functional biomaterials. In: Boccaccini, l.R., Brauer, D.S., Hupa, L. (eds.) Bioactive Glasses: Fundamentals, Technology and Applications, pp. 221–235. Royal Society of Chemistry (2017) Google Scholar
- Cao, W., Hench, L.L.: Bioactive materials. Ceram. Int. 22, 493–507 (1996) ArticleCASGoogle Scholar
- Verné, E., Vitale-Brovarone, C., Bui, E., Bianchi, C.L., Boccaccini, A.R.: Surface functionalization of bioactive glasses. J. Biomed. Mater. Res. 90A, 981–992 (2009) ArticleCASGoogle Scholar
- Kargozar, S., et al.: Functionalization and surface modifications of bioactive glasses (BGs): tailoring of the biological response working on the outermost surface layer. Materials (Basel) 12, 3696 (2019) Google Scholar
- Schuhladen, K., Roether, J.A., Boccaccini, A.R.: Bioactive glasses meet phytotherapeutics: the potential of natural herbal medicines to extend the functionality of bioactive glasses. Biomaterials 217, 119288 (2019) Google Scholar
- Chen, Q.Z., Rezwan, K., Armitage, D., Nazhat, S.N., Boccaccini, A.R.: The surface functionalization of 45S5 Bioglassr-based glass-ceramic scaffolds and its impact on bioactivity. J. Mater. Sci. Mater. Med. 17, 979–987 (2006) ArticleCASGoogle Scholar
- Verné, E., et al.: Surface functionalization of 3D glass–ceramic porous scaffolds for enhanced miner-alization in vitro. Appl. Surf. Sci. 271, 412–420 (2013) ArticleCASGoogle Scholar
- Verné, E., et al.: Alkaline phosphatase grafting on bioactive glasses and glass ceramics. Acta Biomater. 6, 229–240 (2010) ArticleCASGoogle Scholar
- Stanić, S.: Variation in properties of bioactive glasses after surface modification. In: Clinical Applications of Biomaterials, pp. 35–63. Springer, Cham (2017) Google Scholar
- Sun, J., et al.: Functionalization and bioactivity in vitro of mesoporous bioactive glasses. J. Non-Cryst. Solids 354, 3799–3805 (2008) ArticleCASGoogle Scholar
- Gruian, C., Vanea, E., Simon, S., Simon, V.: FTIR and XPS studies of protein adsorption onto functionalized bioactive glass. Biochem. Biophys. Acta. 1824, 873 (2012) CASGoogle Scholar
- Toworfe, G.K., et al.: Effect of functional end groups of silane self-assembled monolayer surfaces on apa-tite formation, fibronectin adsorption and osteoblast cell function. J. Tissue Eng. Regen. Med. 3, 26–36 (2009) ArticleCASGoogle Scholar
- Magyari, K., et al.: Bioactivity evolution of the surface functionalized bioactive glasses. J. Biomed. Mater. Res. Part B 103, 261–272 (2015) ArticleCASGoogle Scholar
- Schickle, K., et al.: Synthesis of novel tricalcium phosphate-bioactive glass composite and functionalization with rhBMP-2. J. Mater. Sci. - Mater. Med. 22, 763–771 (2011) ArticleCASGoogle Scholar
- Massera, J., Mishra, A., Guastella, S., Ferraris, S., Verné, E.: Surface functionalization of phosphate-based bioactive glasses with 3-aminopropyltriethoxysilane (APTS). Biomed. Glass. 2, 51–62 (2016) Google Scholar
- Ferraris, S., Nommeots-Nomm, A., Spriano, S., Vernè, E., Massera, J.: Surface reactivity and silanization ability of borosilicate and Mg-Sr-based bioactive glasses. Appl. Surf. Sci. 475, 43–55 (2019) ArticleCASGoogle Scholar
- Zhang, Y., Luan, J., Jiang, S., Zhou, X., Li, M.: The effect of amino-functionalized mesoporous bioactive glass on MC3T3-E1 cells in vitro stimulation. Composites B 172, 397–405 (2019) ArticleCASGoogle Scholar
- Höhn, S., et al.: Effects of medium pH and preconditioning treatment on protein adsorption on 45S5 bioactive glass surfaces. Adv. Mater. Interf. 7, 2000420 (2020) ArticleCASGoogle Scholar
- Lou, T., Bai, X., He, X., Yuan, C.: Antifouling performance analysis of peptide-modified glass microstructural surfaces. Appl. Surf. Sci. 541, 148384 (2021) Google Scholar
- Zhang, X., et al.: Ultrahigh adhesion force between silica-binding peptide SB7 and glass substrate studied by single-molecule force spectroscopy and molecular dynamic simulation. Front. Chem. 8, 600918 (2020) Google Scholar
- Zhang, L., et al.: Facile surface modification of glass with zwitterionic polymers for improving the blood compatibility. Mater. Res. Exp. 5, 065401 (2018) Google Scholar
- Terpilowski, K., Rymuszka, D.: Surface properties of glass plates activated by air, oxygen, nitrogen and argon plasma. Glass Phys. Chem. 42, 535–541 (2016) ArticleCASGoogle Scholar
- Lopez-Noriega, A., Arcos, D., Vallet-Regí, M.: Functionalizing mesoporous bioglasses for long‐term anti‐osteoporotic drug delivery. Chem. Eur. J. 16, 10879–10886 (2010) Google Scholar
- Leonor, I.B., Alves, C.M., Azevedo, H.S., Reis, R.L.: Effects of protein incorporation on calcium phosphate coating. Mater. Sci. Eng., C 29, 913–918 (2009) ArticleCASGoogle Scholar
- Chen, Q.Z., et al.: Collagen release kinetics of surface functionalized 45S5 Bioglass®-based porous scaffolds. J. Biomed. Mater. Res. Part A 86A, 987–995 (2007) ArticleCASGoogle Scholar
- Zhang, X., Ferraris, S., Prenesti, E., Vernè, E.: Surface functionalization of bioactive glasses with natural molecules of biological significance, part I: gallic acid as model molecule. Appl. Surf. Sci. 287, 329–340 (2013) ArticleCASGoogle Scholar
- Ferraris, S., et al.: Gallic acid grafting to a ferrimagnetic bioactive glass-ceramic. J. Non-Cryst. Solids 432, 167–175 (2016) ArticleCASGoogle Scholar
- Corazzari, I., et al.: Gallic acid grafting modulates the oxidative potential of ferrimagnetic bioactive glass-ceramic SC-45. Colloids Surf. B Biointerf. 148, 592–599 (2016) ArticleCASGoogle Scholar
- Cazzola, M., et al.: Bioactive glasses functionalized with polyphenols: in vitro interactions with healthy and cancerous osteoblast cells. J. Mater. Sci. 52, 9211–9223 (2017) ArticleCASGoogle Scholar
- Cazzola, M., et al.: Bioactive glass coupling with natural polyphenols: surface modification, bioactivity and anti-oxidant ability. Appl. Surf. Sci. 367, 237–248 (2016) ArticleCASGoogle Scholar
- Zhang, X., Ferraris, S., Prenesti, E., Vernè, E.: Surface functionalization of bioactive glasses with natural molecules of biological significance, part II: grafting of polyphenols extracted from grape skin. Appl. Surf. Sci. 287, 341–348 (2013) ArticleCASGoogle Scholar
- Dziadek, M., Dziadek, K., Zagrajczuk, B., Menaszek, E., Cholewa-Kowalska, K.: Poly (“-caprolactone)/bioactive glass composites enriched with polyphenols extract-ed from sage (Salvia officinalis L.). Mater. Lett. 183, 386–390 (2016) Google Scholar
- Sayed Abdelgeliel, A., et al.: Surface functionalization of bioactive glasses with polyphenols from Padina pavonica Algae and in situ reduction of silver ions: physico-chemical characterization and biological response. Coatings 9, 394 (2019) ArticleCASGoogle Scholar
- Ferlenda, G., et al.: Surface functionalization of a silica-based bioactive glass with compounds from Rosa canina bud extracts. ACS Biomater. Sci. Eng. 7, 96–104 (2021) ArticleCASGoogle Scholar
- Malavasi, G., et al.: The role of coordination chemistry in the development of innovative gallium-based bioceramics: the case of curcumin. J. Mater. Chem. 21, 5027–5037 (2011) ArticleCASGoogle Scholar
- Verne, E., et al.: Surface activation of a ferrimagnetic glass-ceramic for antineoplastic drugs graftingy. Adv. Eng. Mater. 12, B309–B319 (2010) Google Scholar
- Zhu, M., Zhang, J., Tao, C., He, X., Zhu, Y.: Design of mesoporous bioactive glass/hydroxyapatite composites for controllable co-delivery of chemotherapeutic drugs and proteins. Mater. Lett. 115, 194–197 (2014) ArticleCASGoogle Scholar
- Boanini, E., et al.: Alendronate functionalized mesoporous bioactive glass nanospheres. Materials (Basel) 9, 135 (2016) Google Scholar
- Aina, V., et al.: New formulation of functionalized bioactive glasses to be used as carriers for the development of pH-stimuli responsive biomaterials for bone diseases. Langmuir 30, 4703–4715 (2014) ArticleCASGoogle Scholar
- Ferraris, S., et al.: Effects of sterilization and storage on the properties of ALP-grafted biomaterials for prosthetic and bone tissue engineering applications. Biomed. Mater. 7, 054102 (2012) Google Scholar
- Jones, J.R., Brauer, D.S., Greenspan, D.C.: Bioglass and bioactive glasses and their impact on healthcare. Int. J. Appl. Glas. Sci. 7, 423–432 (2016) ArticleCASGoogle Scholar
- Merwin, G.E.: Bioglass middle ear prosthesis: preliminary report. Ann. Otol. Rhinol. Laryngol. 95, 78–82 (1986) ArticleCASGoogle Scholar
- Bahmad, F., Merchant, S.N.: Histopathology of ossicular grafts and implants in chronic otitis media. Ann. Otol. Rhinol. Laryngol. 116, 181–191 (2007) ArticleGoogle Scholar
- Downing, M., et al.: A bone-anchored percutaneous connector system for neural prosthetic applications. Ear Nose Throat J. 76, 328–332 (1997) ArticleCASGoogle Scholar
- Stanley, H., Hall, M., Clark, A.: Using 45S5 bioglass cones as endosseous ridge maintenance implants to prevent alveolar ridge resorption: a 5-year evaluation. Int. J. Oral Maxillofac. Implants 12, 1–19 (1997) Google Scholar
- Baino, F.: Ceramics for bone replacement: commercial and clinical use. In: Palmero, P., Cambier, F., De Barra, E. (eds.) Advances in Ceramic Biomaterials, pp. 249–278. Woodhead Publishing, Elsevier (2017) Google Scholar
- Chen, Q.Z., Thompson, I.D., Boccaccini, A.R.: 45S5 Bioglass®-derived glass–ceramic scaffolds for bone tissue engineering. Biomaterials 27, 2414–2425 (2006) ArticleCASGoogle Scholar
- Gillam, D.G., Tang, J.Y., Mordan, N.J., Newman, H.N.: The effects of a novel Bioglass® dentifrice on dentine sensitivity: a scanning electron microscopy investigation. J. Oral Rehabil. 29, 305–313 (2002) ArticleCASGoogle Scholar
- Vahid Golpayegani, M., Sohrabi, A., Biria, M., Ansari, G.: Remineralization effect of topical NovaMin versus sodium fluoride (1.1%) on caries-like lesions in permanent teeth. J. Dentistry (Tehran) 9, 68–75 (2012) Google Scholar
- Banerjee, A., Hajatdoost-Sani, M., Farrell, S., Thompson, I.: A clinical evaluation and comparison of bioactive glass and sodium bicarbonate air-polishing powders. J. Dent. 38, 475–479 (2010) ArticleCASGoogle Scholar
- Kargozar, S., Baino, F., Hamzehlou, S., Hill, R.G., Mozafari. M.: Bioactive glasses: sprouting angiogenesis in tissue engineering. Trends Biotechnol. 36, 430–444 (2018) Google Scholar
- Wray, P.: Cotton candy’that heals. Am. Ceram. Soc. Bull. 90, 24–31 (2011) CASGoogle Scholar
- Ma, X., Schou, K.R., Maloney-Schou, M., Harwin, F.M., Ng, J.D.: The porous polyethylene/bioglass spherical orbital implant: a retrospective study of 170 cases. Ophthalmic Plast. Reconstr. Surg. 27, 21–27 (2011) ArticleCASGoogle Scholar
- Miola, M., et al.: Glass-ceramics for cancer treatment: so close, or yet so far? Acta Biomater. 83, 55–70 (2019) ArticleCASGoogle Scholar
- Velasco, M.V., Souza, M.T., Crovace, M.C., Aparecido de Oliveira, A.J.A., Zanotto, E.D.: Bioactive magnetic glass-ceramics for cancer treatment. Biomed. Glass. 5, 148–177 (2019) Google Scholar
- Cochis, A., Miola, M., Bretcanu, O., Rimondini, L., Verné, E.: Magnetic bioactive glass ceramics for bone healing and hyperthermic treatment of solid tumors. In: Tiwari, A., Iyer, P. K., Kumar, V., Swart, H. (eds.) Advanced Magnetic and Optical Materials, pp. 81–112. Scrivener Publishing LLC (2016) Google Scholar
- Miola, M., et al.: Composite bone cements for hyperthermia: modeling and characterization of magnetic, calorimetric and in vitro heating properties. Ceram. Int. 43, 4831–4840 (2017) ArticleCASGoogle Scholar
- Bretcanu, O., et al.: In vitro biocompatibility of a ferrimagnetic glass-ceramic for hyperthermia application. Mater. Sci. Eng., C 73, 778–787 (2017) ArticleCASGoogle Scholar
- Miola, M., Gerbaldo, R., Laviano, F., Bruno, M., Vernè, E.: Multifunctional ferrimagnetic glass–ceramic for the treatment of bone tumor and associated complications. J. Mater. Sci. 52, 9192–9201 (2017) ArticleCASGoogle Scholar
- Verné, E., et al.: Composite bone cements loaded with a bioactive and ferrimagnetic glass-ceramic: leaching, bioactivity and cytocompatibility. Mater. Sci. Eng., C 53, 93–103 (2015) ArticleCASGoogle Scholar
- Tiberto, P., et al.: Magnetic relaxation in ferrimagnetic glass-ceramics obtained by co-precipitation at different temperatures. IEEE Trans. Magn. 43, 2471–2473 (2007) ArticleCASGoogle Scholar
- Bretcanu, O., Verné, E., Coisson, M., Tiberto, P., Allia, P.: Temperature effect on the magnetic properties of the coprecipitation-derived ferrimagnetic glass-ceramics. J. Magn. Magn. Mater. 300, 412–417 (2006) ArticleCASGoogle Scholar
- Bretcanu, O., Verné, E., Coisson, M., Tiberto, P., Allia, P.: Magnetic properties of the ferromagnetic glass-ceramics for hyperthermia. J. Magn. Magn. Mater. 305, 529–533 (2006) ArticleCASGoogle Scholar
- Bretcanu, O., Spriano, S., Verné, E., Coisson, M., Tiberto, P.: The influence of crystallised Fe3O4 on the magnetic properties of coprecipita-tion-derived ferrimagnetic glass-ceramics. Acta Biomater. 1, 421–429 (2005) Google Scholar
- Bretcanu, O., Spriano, S., Vitale-Brovarone, C., Verné, E.: Synthesis and characterization of coprecipitation-derived ferrimagnetic glass-ceramics. J. Mater. Sci. 41, 1029–1037 (2006) ArticleCASGoogle Scholar
- Day, D.E.: Glasses for radiotherapy. In: Jones, J.R., Clarke, G.C. (eds.) Bio-Glasses: An Introduction, pp. 203–228. Wiley (2012) Google Scholar
- Miguez-Pacheco, V., Hench, L.L., Boccaccini, A.R.: Bioactive glasses beyond bone and teeth: emerging applications in contact with soft tissues. Acta Biomater. 13, 1–15 (2015) ArticleCASGoogle Scholar
- Baino, F., Novajra, G., Miguez-pacheco, V., Boccaccini, A.R., Vitale-Brovarone, C.: Bioactive glasses: special applications outside the skeletal system. J. Non-Cryst. Solids 432, 15–30 (2016) ArticleCASGoogle Scholar
- Kargozar, S., Hamzehlou, S., Baino, F.: Potential of bioactive glasses for cardiac and pulmonary tissue engineering. Materials (Basel). 10, 1429 (2017) Google Scholar
- Gocha, A., McDonald, L.: Better bodies with biomaterials: how ceramic and glass contribute to the $110B global market for implantable bio-materials. Am. Ceram. Soc. Bull. 99, 17–31 (2020) CASGoogle Scholar
Author information
Authors and Affiliations
- Department of Applied Science and Technology, Institute of Materials Physics and Engineering, Politecnico Di Torino, Corso Duca degli Abruzzi 24, 10129, Torino, Italy Francesco Baino, Carla Migneco, Elisa Fiume, Marta Miola, Sara Ferraris, Silvia Spriano, Monica Ferraris & Enrica Verné
- Francesco Baino